Chihiro Iwaya, PhD
Research Associate
[email protected]
713-486-2678
Visit Profile
Overview
Role of cellular metabolism during development and diseases
Cellular metabolic aberrations result in craniofacial deformities in humans and mice. Interestingly, mice with cholesterol synthesis deficiency have severe malformations specifically in the craniofacial region and the rest of their body is largely unaffected. The majority of cells in craniofacial region are derived from cranial neural crest (CNC) cells, which is a multi-potent cell population that gives rise to a variety of different cell types. These results suggest that CNC cells are more sensitive to cellular metabolic aberrations than are cells from other regions during embryogenesis. However, it is still unknown how molecules related to cellular metabolism are regulated during craniofacial development. In addition, the possible relationship between the cellular metabolism and craniofacial deformities remain unclear. The aim of our laboratory is to identify gene mutations and protein modifications related to craniofacial disorders and provide the basis for tests aimed at identifying higher-risk persons.
Role of autophagic machinery in development and diseases
Autophagy is an evolutionarily conserved bulk-protein degradation system, in which isolation membranes engulf cytoplasmic constituents and the resulting autophagosomes transport them to lysosomes, crucial for the removal and breakdown of cellular components such as damaged proteins and aged organelles. Autophagic activity is altered in various diseases and birth defects in humans and mice. An understanding of the manner in which autophagy is regulated is critical for understanding normal craniofacial development as well as congenital malformations. The aim of our laboratory is to identify the molecular regulatory mechanism of autophagic machinery related to developmental defects and diseases.
Projects
-
Bone study
Bone study
Human linkage studies have shown that genetic mutations related to cholesterol metabolism and high maternal cholesterol diets result in craniofacial bone deformities, suggesting that cholesterol metabolic aberrations may be a widely conserved mechanism in craniofacial developmental defects. However, it is still largely unknown how altered cholesterol metabolism causes craniofacial bone abnormalities. Therefore, the objective of our study is to define how cholesterol metabolic aberrations cause osteoblast and osteoclast differentiation abnormalities, and to test the functional significance of downstream target molecules during craniofacial bone formation and homeostasis. The long-term goal of this project is to gain insight into the mechanisms of craniofacial bone formation and homeostasis and provide new medications and diagnostic tools for bone diseases.
-
Salivary Gland Study
Salivary Gland Study
Sjögren’s syndrome (SS) is a chronic autoimmune disease characterized by immune cell infiltration of the exocrine glands, mainly the salivary and lacrimal glands. The differential diagnosis and etiology of SS is extensive since there are several diseases with similar symptoms and various factors associated with SS. The current diagnostic criteria for SS consists in a combination of histological examinations (biopsy) of minor SGs for immune cell foci, serology for autoantibodies, and dry eye/mouth evaluation; therefore, there is no single test that will confirm a diagnosis for SS. Since patients are diagnosed only at later stages of disease, the earlier events prior to clinical manifestations are largely unknown. Taking advantage of our newly developed SS animal models, we aim to determine how disruption in exocytosis leads to SS and how excessive cholesterol synthesis in salivary gland tissues leads to SS. A detailed understanding of the mechanism(s) will provide new knowledge of the mechanisms of SS. Ultimately, this study will identify novel targets for therapeutics and new diagnostic tools for identifying SS at an early pathological stage in at-risk populations.
-
Muscle Study
Muscle Study
Adult regenerative myogenesis is vital for restoring normal tissue structure after muscle injury. Muscle repair is dependent on progenitor satellite cells, which proliferate in response to injury, and their progeny differentiate and undergo cell-cell fusion to form regenerating myofibers. Myogenic progenitor cells must be precisely regulated and positioned for proper cell fusion to occur. We previously reported that WNT signaling is regulated in a spatiotemporal specific manner during muscle development (Mol Cell Biol, 2015; Sci Rep, 2018). We are further investigating how this signaling pathway regulates muscle development and regeneration. Our long-term goal is to better understand the mechanisms of muscle development and repair, to develop therapeutic interventions for muscle injury and diseases/disorders, and to devise potential strategies to prevent muscle developmental defects and accelerate muscle regeneration.
-
Cleft Lip/Cleft Palate Study
Cleft Lip/Cleft Palate Study
Cleft palate is one of the most common congenital birth defects, with a prevalence of 1/700 live births worldwide. The etiology of cleft lip and palate is complicated by a variety of genetic and environmental factors. This study will identify the distribution and contribution of non-coding RNAs (ncRNAs) in lip and palate development. We hypothesize that various environmental factors and metabolic aberrations that cause cleft lip and palate through expression of ncRNAs that suppress the expression and activity of genes associated with craniofacial anomalies. The results of this study will enhance our understanding of the role of ncRNAs in lip and palate development and will enable us to design future therapeutic approaches to diagnose and prevent cleft lip and palate.
Human linkage studies have shown that either genetic mutations related to cholesterol metabolism or abnormal maternal cholesterol diets lead to craniofacial deformities such as cleft palate. However, it is largely unknown how disturbances in cholesterol production result in cleft palate. For instance, mutations in genes involved in cholesterol synthesis (SC5D, DHCR7, and DHCR24) have been found in lathosterolosis, Smith-Lemli-Opitz Syndrome [SLOS], and desmosterolosis. Patients with these syndromes display craniofacial abnormalities (e.g. cleft palate) with a wide array of degree in severity. In addition, high-cholesterol diets during pregnancy are known to be a risk factor for birth defects, including various craniofacial abnormalities. Sterol-C5-desaturase (SC5D) catalyzes the dehydrogenation of a C-5(6) bond in a sterol intermediate during compound cholesterol biosynthesis. Mutations in SC5D result in cholesterol deficiency as well as in excessive lathosterol, a cholesterol precursor that causes lathosterolosis, which is characterized by craniofacial deformities such as cleft palate, dysmorphism and micrognathia, and limb anomalies. By contrast, mutations in DHCR7, which encodes 7-dehydrocholesterol reductase, lead to SLOS, which is characterized by less severe craniofacial deformities (e.g. high-arched palate, less frequent cleft palate, ptosis, a short nasal root, etc.) compared with lathosterolosis. These findings suggest that accumulated cholesterol precursors lathosterol and 7-dehydrocholesterol differently interfere with the process of craniofacial development. Thus, while proper regulation of cholesterol metabolism is crucial for craniofacial development, the exact mechanism of how cholesterol metabolism affects craniofacial development remains largely unknown. The results of this study will enhance our understanding of the role of cholesterol metabolism in lip and palate development and will enable us to design future therapeutic approaches to diagnose and prevent cleft lip and palate. -
Tooth Development Study
Tooth Development Study
Inherited tooth enamel defects (a.k.a. amelogenesis imperfecta) occur worldwide with a prevalence of 0.5%. Major clinical impacts include social embarrassment, dental pain, eating difficulties, and early tooth loss due to abnormally thin, soft, fragile, and discolored enamel, together with poor aesthetics and functionality. Although amelogenesis imperfecta is a significant and urgent dental issue, the etiology of amelogenesis imperfecta remains largely unknown owing to potential complex genetic interactions. We have recently reported that microRNAs (miRNAs), short non-coding RNAs that regulate expression of target genes at post-transcriptional level, play a crucial role in the regulation of genes associated with ameloblast differentiation in humans and mice. In addition, we found that mice with autophagy deficiency displayed amelogenesis imperfecta due to a failure in ameloblast differentiation. Although an increasing number of studies indicate that autophagy-related molecules play important roles in various cellular processes, including transcriptional regulation in physiological and pathological conditions, the transcriptional mechanism regulating differentiation remains unclear. This study will identify a novel mechanism for amelogenesis imperfecta and help develop diagnostic tools and therapeutic interventions in at-risk populations and families.
Tooth agenesis (a.k.a. missing teeth) occurs with a prevalence of approximately 2–10% (excluding the 3rd molars) worldwide; a missing 3rd molar is more common, occurring at a rate of approximately 20% worldwide. Although tooth agenesis is a significant and urgent dental issue, the etiology of tooth agenesis remains largely unknown owing to potential complex genetic interactions. As of today, human genetic studies have identified genetic susceptibility to tooth agenesis in various populations and ethnic groups. Although recent technological advancements have contributed to the discovery of a number of novel genetic mutations associated with tooth agenesis, the regulatory network of these genes remains mostly unclear. Interestingly, several cholesterol metabolic syndromes are characterized by dental deformities; however, it remains unclear how altered cholesterol metabolism leads to dental abnormalities. The objective of this study is to define how cholesterol metabolic aberrations cause dental abnormalities, and to test the functional significance of downstream target molecules during tooth formation.
Lab Team
Kanwarjot Singh, B.S.
Staff Research Assistant
[email protected]
713-486-2678
Visit Profile
Yasmeen Ghassan Dweik, BDS
Staff Research Assistant
[email protected]
713-486-2678
Visit Profile
Aemin Kim
UTSD Dental Student
[email protected]
Visit Profile
Sunny Yu
Visiting Student, Rice University
[email protected]
Visit Profile
Key Publications
Original Articles in Journals:
1. Schuler R, Bugacov A, Hacia J, Hou T, Iwata J, Pearlman L, Samuels B, Williams C, Zhao Z, Kesselman C, and Chai Y (2022) FaceBase: a community-driven Hub for data intensive research. J. Dent. Res. 2022 Oct;101(11):1289-1298. PMID: 35912790.
2. Yan F, Simon LM, Suzuki A, Iwaya C, Jia P, Iwata J#, and Zhao Z# (2022) Spatiotemporal microRNA-gene expression network in craniofacial development. J. Dent. Res. 2022 Oct;101(11):1398-1407. PMID: 35774010. #: Corresponding authors.
3. Suzuki A, Iwaya C, Ogata K, Yoshioka H, Shim J, Tanida I, Komatsu M, Tada N, and Iwata J (2022) Impaired GATE16-mediated exocytosis in exocrine tissues causes Sjögren’s syndrome-like exocrinopathy. Cell. Mol. Life Sci. 2022 May 20;79(6):307. PMID: 35593968.
4. Shim J, Iwaya C, Ambrose CG, Suzuki A, and Iwata J (2022) Micro-computed tomography assessment of bone structure in aging mice. Scientific Reports. 2022 May 17;12(1):8117. PMID: 35581227.
5. Yoshioka H, Suzuki A, Iwaya C, and Iwata J (2022) Suppression of microRNA-124-3p and microRNA-340-5p ameliorates retinoic acid-induced cleft palate in mice. Development. 2022 Apr 14:dev.200476. PMID: 35420127.
6. Suzuki A, Yoshioka H, Liu T, Gull A, Singh N, Le T, Zhao Z, and Iwata J (2022) Crucial roles of microRNA-16-5p and microRNA-27b-3p in ameloblast differentiation through regulation of genes associated with amelogenesis imperfecta. Frontiers in Genetics. 2022 Mar 25;13:788259. PMID: 35401675.
7. Kanazawa S, Okada H, Riu D, Mabuchi Y, Akazawa C, Iwata J, Hoshi K, and Hikita A (2022) Hematopoietic-mesenchymal signals regulate the properties of mesenchymal stem cells. International Journal of Molecular Sciences. 2022 Jul 26;23(15):8238. PMID: 35897814.
8. Yoshioka H, Jun G, Suzuki A, and Iwata J (2021) Dexamethasone suppresses palatal cell proliferation through miR-130a. International Journal of Molecular Sciences. 2021 Nov 18;22(22):12453. PMID: 34830336.
9. Xu H, Yan F, Hu R, Suzuki A, Iwaya C, Jia P, Iwata J#, and Zhao Z# (2021) CleftGeneDB: A resource for annotating genes associated with cleft lip and cleft palate. Science Bulletin. Volume 66, Issue 23;2340-2342. DOI : https://doi.org/10.1016/j.scib.2021.07.008. #: Corresponding authors.
10. Yoshioka H, Li A, Suzuki A, Ramakrishnan SS, Zhao Z, and Iwata J (2021) Identification of microRNAs and gene regulatory networks in cleft lip common in humans and mice. Human Molecular Genetics. 2021 Sep 15;30(19): 1881-93. PMID: 34104955.
11. Yoshioka H, Mikami Y, Ramakrishnan SS, Suzuki A, and Iwata J (2021) MicroRNA-124-3p plays a crucial role in cleft palate induced by retinoic acid. Frontiers in Cell and Developmental Biology. 2021 Jun 9;9:621045. PMID: 34178974.
12. Yoshioka H, Wang Y, Suzuki A, Shayegh M, Gajera M, Zhao Z, and Iwata J (2021) Overexpression of miR-1306-5p, miR-3195, and miR-3914 inhibits ameloblast differentiation through suppression of genes associated with human amelogenesis imperfecta. International Journal of Molecular Sciences. 2021 Feb 23;22(4):2202. PMID: 33672174.
13. Yoshioka H, Ramakrishnan SS, Suzuki A, and Iwata J (2021) Phenytoin inhibits cell proliferation through microRNA-196a-5p in mouse lip mesenchymal cells. International Journal of Molecular Sciences. 2021 Feb 9;22(4):1746. PMID: 33572377.
14. Yoshioka H, Ramakrishnan SS, Shim J, Suzuki A, and Iwata J (2021) Excessive all-trans retinoic acid inhibits cell proliferation through upregulated microRNA-4680-3p in cultured human palate cells. Frontiers in Cell and Developmental Biology. 2021 Jan 28;9:618876. PMID: 33585479.
15. Kanazawa S, Okada H, Hojo H, Ohba S, Iwata J, Komura M, Hikita A, and Hoshi K (2021) Mesenchymal stromal cells in the bone marrow niche consist of multi-populations with distinct transcriptional and epigenetic properties. Scientific Reports. 2021 Aug 4;11(1):15811. PMID: 34349154.
16. Yan F, Jia P, Yoshioka H, Suzuki A, Iwata J#, and Zhao Z# (2020) A developmental stage specific network approach for studying dynamic transcription factor-microRNA co-regulation during craniofacial development. Development. 2020 Dec 24;147(24):dev192948. PMID: 33234712. #: Corresponding authors.
17. Yang Y, Suzuki A, Iwata J, and Jun G (2020) Secondary Genome-Wide Association Study using novel analytical strategies disentangle genetic components of cleft lip and/or cleft palate in 1q32.2. Genes. 2020 Oct 29;11(11):1280. PMID: 33137956.
18. Suzuki A, Ogata K, Yoshioka H, Shim J, Wassif CA, Porter FD, and Iwata J (2020) Cholesterol metabolism regulates bone formation and homeostasis through primary cilium formation. Bone Research. Jan 2;8:1. PMID: 31934493.
19. Yan F, Dai Y, Iwata J, Zhao Z, and Jia P (2020) An integrative, genomic, transcriptomic and network-assisted study to identify genes associated with human cleft lip with or without cleft palate. BMC Medical Genomics. 13(Suppl 5):39, PMID: 32241273.
20. Suzuki A, Yoshioka H, Summakia D, Desai NG, Jun G, Jia P, Loose DS, Ogata K, Gajera MV, Zhao Z, and Iwata J (2019) MicroRNA-124-3p suppresses mouse lip mesenchymal cell proliferation through the regulation of genes associated with cleft lip in the mouse. BMC Genomics. Nov 14;20(1):852. PMID: 31727022.
21. Suzuki A, Shim J, Ogata K, Yoshioka H, and Iwata J (2019) Cholesterol metabolism plays a crucial role in the regulation of autophagy for cell differentiation of granular convoluted tubules in male mouse submandibular glands. Development. Oct 17;146(20). PMID: 31558435.
22. Li A, Jia P, Malik S, Fei R, Yoshioka H, Suzuki A, Iwata J, and Zhao Z (2019) Critical microRNAs and regulatory motifs in cleft palate identified by a conserved microRNA-TF-gene network approach in humans and mice. Briefings in Bioinformatics. Oct 7, PMID: 31589286.
23. Gajera M, Desai N, Suzuki A, Li A, Zhang M, Jun G, Jia P, Zhao Z, and Iwata J (2019) MicroRNA-655-3p and microRNA-497-5p inhibit cell proliferation in cultured human lip cells through the regulation of genes related to human cleft lip. BMC Medical Genomics. May 23;12(1):70. PMID: 31122291.
24. Suzuki A, Li A, Gajera M, Abdallah N, Zhang M, Zhao Z, and Iwata J (2019) MicroRNA-374a, -4680, and -133b suppress cell proliferation through the regulation of genes associated with human cleft palate in cultured human palate cells. BMC Medical Genomics. Jul 1;12(1):93. PMID: 31262291.
25. Li A, Qin G, Suzuki A, Gajera M, Iwata J, Jia P, and Zhao Z (2019) Network-based identification of critical regulators as putative drivers of human cleft lip. BMC Medical Genomics. 2019, Jan 31;12(Suppl 1):16. PMID: 30704473.
26. Suzuki A, Minamide R, and Iwata J (2018) The role of acetyltransferases for the temporal-specific accessibility of β-catenin to the myogenic gene locus. Scientific Reports. Oct 10;8(1):15057. PMID: 30305648.
27. Suzuki A, Minamide R, and Iwata J (2018) WNT/β-catenin signaling plays a crucial role in myoblast fusion through the regulation of Nephrin expression during development. Development. Nov 27;145(23). PMID: 30389854.
28. Suzuki A, Jun G, Abdallah N, Gajera M, and Iwata J (2018) Gene datasets associated with mouse cleft palate. Data in Brief. Mar 14;18:655-673. PMID: 29896534.
29. Suzuki A, Abdallah N, Gajera M, Jun G, Jia P, Zhao Z, and Iwata J (2018) Genes and microRNAs associated with mouse cleft palate: a systematic review and bioinformatics analysis. Mechanisms of Development. Apr;150:21-27. PMID: 29475039.
30. Carlock C, Wu J, Shim J, Moreno-Gonzalez I, Pitcher MR, Hicks J, Suzuki A, Iwata J, Quevado J, and Lou Y (2017) Interleukin33 deficiency causes tau abnormality and neurodegeneration with Alzheimer-like symptoms in aged mice. Transl. Psychiatry. Jul 4;7(7):e1164. PMID: 28675392.
31. Suzuki A, Pelikan RC, and Iwata J (2015) WNT/β-catenin signaling regulates multiple steps of myogenesis by regulating step-specific targets. Mol. Cell. Biol. May 15; 35 (10): 1763-76. PMID: 25755281.
32. Ho TV*, Iwata J*, Ho HA, Grimes WC, Park S, Sanchez-Lara PA, and Chai Y (2015) Integration of comprehensive 3D microCT and signaling analysis reveals differential regulatory mechanisms of craniofacial bone development. Dev. Biol. Apr 15; 400 (2): 180-90. *These authors contributed equally to this work.
33. Iwata J, Suzuki A, Yokota T, Ho TV, Pelikan RC, Urata M, Sanchez-Lara P, and Chai Y (2014) TGFβ regulates epithelial–mesenchymal interactions through WNT signaling activity to control muscle development in the soft palate. Development, Feb; 141 (4): 909-17.
34. Iwata J, Suzuki A, Pelikan RC, Ho TV, Sanchez-Lara PA, and Chai Y (2014) Modulation of lipid metabolic defects rescues cleft palate in Tgfbr2 mutant mice. Hum. Mol. Genetics, Jan 1; 23 (1): 182-93.
35. Iwata J, Suzuki A, Pelikan RC, Ho TV, and Chai Y (2013) Cranial neural crest cells regulate tongue muscle formation via TGFβ–mediated BMP and FGF signaling. J. Biol. Chem. 288: 29760-70.
36. Parada C, Li J, Iwata J, Suzuki A, and Chai Y (2013) CTGF mediates Smad-dependent transforming growth factor β signaling to regulate mesenchymal cell proliferation during palate development. Mol. Cell Biol., Sep; 33 (17): 3482-93.
37. Koike M, Tanida I, Nanao T, Tada N, Iwata J, Ueno T, Kominami E, and Uchiyama Y (2013) Enrichment of GABARAP relative to LC3 in the axonal initial segments of neurons. PLoS ONE, May 9; 8 (5): e63568.
38. Song ZC*, Liu C*, Iwata J*, Gu SP, Suzuki A, Sun C, He W, Shu R, Li L, Chai Y, and Chen YP (2013) Mice with Tak1-deficiency in neural crest lineage exhibit cleft palate associated with abnormal tongue development. J. Biol. Chem., Apr 12; 288 (15): 10440-50. (*These authors contributed equally to this work.)
39. Iwata J, Suzuki A, Pelikan RC, Ho TV, Sanchez-Lara PA, Urata M, Dixon MJ, and Chai Y (2013) Smad4–Irf6 genetic interaction and TGFβ–mediated IRF6 signaling cascade are crucial for palatal fusion in mice. Development, Mar; 140 (6): 1220-30.
40. Pelikan RC*, Iwata J*, Suzuki A, Chai Y, and Hacia JG (2013) Identification of candidate downstream targets of TGFβ signaling during palate development by genome-wide transcript profiling. J. Cell Biochem., Apr, 114: 796-807. (*These two authors contributed equally to this work.)
41. Iwata J, Hacia JG, Suzuki A, Sanchez-Lara PA, Urata M, and Chai Y (2012) Modulation of non-canonical TGF-β signaling prevents cleft palate in Tgfbr2 mutant mice. J. Clin. Invest., Mar 1; 122 (3): 873-85.
42. Iwata J, Tung L, Urata M, Hacia JG, Suzuki A, Ramenzoni L, Chaudhry O, Parada C, Sanchez-Lara PA, and Chai Y (2012) Fibroblast growth factor 9 (FGF9)–pituitary homeobox 2 (PITX2) pathway mediates transforming growth factor beta (TGFβ) signaling to regulate cell proliferation in palatal mesenchyme during mouse palatogenesis. J. Biol. Chem., Jan 20; 287 (4): 2353-63.
43. Okamoto K, Okamoto Y, Kawakubo T, Iwata J, Yasuda Y, Tsukuba T, and Yamamoto K (2011) Role of the transcription factor Sp1 in regulating the expression of the murine cathepsin E gene. J. Biochem., Nov 30; 151 (3): 263-72.
44. Hochheiser H, Aronow BJ, Artinger K, Beaty TH, Brinkley JF, Chai Y, Clouthier D, Cunningham ML, Dixon M, Donahue LR, Fraser SE, Iwata J, Marazita ML, Murray JC, Murray S, Postlethwait J, Potter S, Shapiro L, Spritz R, Visel A, Weinberg SM, Trainor PA (2011) The FaceBase Consortium: A comprehensive program to facilitate craniofacial research. Dev. Biol., Jul 15; 355 (2): 175-182.
45. Huang XF, Yokota T, Iwata J, Chai Y (2011) TGF-β mediated FasL–Fas–Caspase pathway is crucial during palatogenesis. Journal of Dental Research, Aug; 90 (8): 981-987.
46. Ezaki J, Matsumoto N, Takeda-Ezaki M, Komatsu M, Takahashi K, Hiraoka Y, Taka H, Fujimura T, Takehana K, Yoshida M, Iwata J, Tanida I, Furuya N, Zheng D, Tada N, Tanaka K, Kominami E, and Ueno T (2011) Liver autophagy contributes to the maintenance of blood glucose and amino acid levels. Autophagy, Jul; 7 (7): 727-736.
47. Chung IH, Han J, Iwata J, and Chai Y (2010) Msx1 and Dlx5 function synergistically to regulate frontal bone development. Genesis, Vol. 48 (11) 645-655.
48. Iwata J, Hosokawa R, Sanchez-Lara PA, Urata M, Slavkin H, and Chai Y (2010) Transforming growth factor-beta regulates basal transcriptional regulatory machinery to control cell proliferation and differentiation in cranial neural crest–derived osteoprogenitor cells. J. Biol. Chem., Feb 12; 285 (7): 4975-82.
49. Hosokawa R, Oka K, Yamaza T, Iwata J, Urata M, Xu X, Bringas P Jr, Nonaka K, and Chai Y (2010) TGF-β mediated FGF10 signaling in cranial neural crest cells controls development of myogenic progenitor cells through tissue–tissue interactions during tongue morphogenesis. Dev. Biol., 341 (1): 186-95.
50. Sou Y*, Waguri S*, Iwata J*, Ueno T, Fujimura T, Hara T, Sawada N, Yamada A, Mizushima N, Uchiyama Y, Kominami E, Tanaka K, Komatsu M (2008). The Atg8 conjugation system is indispensable for proper development of autophagic isolation membranes in mice. Mol. Biol. Cell, 19 (11); 4762-75. *These three authors contributed equally to this work.
51. Komatsu M, Waguri S, Koike M, Sou Y, Ueno T, Hara T, Mizushima N, Iwata J, Ezaki J, Murata S, Hamazaki J, Nishito Y, Iemura S, Natsume T, Yanagawa T, Uwayama J, Warabi E, Yoshida H, Ishii T, Kobayashi A, Yamamoto M, Yue Z, Uchiyama Y, Kominami E, and Tanaka K (2007). Homeostatic levels of p62 control cytoplasmic inclusion body formation in autophagy-deficient mice. Cell, 131, 1149-1163.
52. Kawakubo T, Okamoto K, Iwata J, Shin M, Okamoto Y, Yasukochi A, Nakayama K, Kadowaki T, Tsukuba T, and Yamamoto K (2007). Cathepsin E prevents tumor growth and metastasis by catalyzing the proteolytic release of soluble TRAIL from tumor cell surface. Cancer Research, 67 (22): 10869-78.
53. Shin M, Kadowaki T, Iwata J, Kawakubo T, Yamaguchi N, Takii R, Tsukuba T, and Yamamoto K (2007). Association of cathepsin E with tumor growth arrest through angiogenesis inhibition and enhanced immune responses. Biol. Chem., 388 (11): 1173-81.
54. Komatsu M, Wang QJ, Holstein GR, Friedrich VL Jr, Iwata J, Kominami E, Chait BT, Tanaka K, and Yue Z (2007). Essential role for autophagy protein Atg7 in the maintenance of axonal homeostasis and the prevention of axonal degeneration. Proc. Natl. Acad. Sci. USA, Sep 4; 104 (36): 14489-94.
55. Komatsu M, Waguri S, Chiba T, Murata S, Iwata J, Tanida I, Ueno T, Koike M, Uchiyama Y, Kominami E, and Tanaka K (2006). Loss of autophagy in the central nervous system causes neurodegeneration. Nature, 441 (7095), 880-884.
56. Iwata J, Ezaki J, Komatsu M, Yokota S, Ueno T, Tanida I, Chiba T, Tanaka K, and Kominami E (2006) Excess peroxisomes are degraded by autophagic machinery in mammals. J. Biol. Chem., 281, 4035-41.
57. Komatsu M, Waguri S, Ueno T, Iwata J, Murata S, Tanida I, Ezaki J, Mizushima N, Ohsumi Y, Uchiyama Y, Kominami E, Tanaka K, and Chiba T (2005) Impairment of starvation–induced and constitutive autophagy in Atg7–deficient mice. J. Cell Biol., 169, 425-434.
Refereed Review Articles:
1. Iwaya C, Suzuki A, and Iwata J (2023) MicroRNAs and gene regulatory networks related to cleft lip and palate. International Journal of Molecular Sciences. 2023,24(4),3552.
2. Suzuki A and Iwata J (2021) Amino acid metabolism and autophagy in skeletal development and homeostasis. Bone. 2021 May;146:115881. PMID: 33578033.
3. Suzuki A, Ogata K, and Iwata J (2021) Cell signaling regulation in salivary gland development. Cellular and Molecular Life Sciences. 2021 Jan 15. PMID: 33449148.
4. Suzuki A, Minamide M, Iwaya C, Ogata K, and Iwata J (2020) Role of metabolism in bone development and homeostasis. International Journal of Molecular Sciences. 2020 Nov 26;21(23):8992. PMID: 33256181. This review article was selected as one of the Top Downloaded Paper of IJMS in 2020.
5. Iwata J (2020) Gene ? environment interplay and microRNAs in cleft lip and cleft palate. Oral Science International (2020).
6. Iwata J (2020) Regulatory mechanism of salivary protein secretion. Japanese Association of Oral Science (2020).
7. Suzuki A and Iwata J (2018) Molecular regulatory mechanism of exocytosis in the salivary glands. International Journal of Molecular Sciences. 2018 Oct 17;19(10):3208. PMID: 30336591.
8. Suzuki A, Sangani DR, Ansari A, and Iwata J (2016) Molecular mechanism of midfacial developmental defects. Developmental Dynamics, Mar;245(3):276-93. PMID: 26562615.
9. Suzuki A and Iwata J (2016) Mouse genetic models for temporomandibular joint development and disorders. Oral Diseases, Jan;22(1):33-38. PMID: 26096083.
10. Sangani D, Suzuki A, VonVille H, Hixson JE, and Iwata J (2015) Gene mutations associated with temporomandibular joint disorders: a systematic review. Open Access Library Journal, Doi:10.4236/oalib.1101583.
11. Suzuki A and Iwata J (2015) WNT signaling mechanism in muscle development and homeostasis. Journal of Nature and Science. 2015; 1 (8): e143.
12. Suzuki A, Sangani DR, and Iwata J (2014) Molecular mechanism of cranial neural crest cell development. Journal of Dentistry and Clinical Research, October, 1 (1): 005.
13. Suzuki A and Iwata J (2014) Genetic and environmental risk factors for cleft lip and cleft palate. Progressive Science, 1:e01. doi: 10.14721/pscience.2014.e01.
14. Iwata J, Parada C, and Chai Y. (2011) The mechanism of TGF-β signaling during palate formation: a novel target for prevention of cleft palate. Oral Diseases, Nov; 17 (8): 733-744.
15. Iwata J, Kawakubo T, and Yamamoto K. (2004) Angiostatin. Connective Tissue, 36 (3), 165-169.
Invited Review Articles:
1. Iwata J and Yamamoto K. (2004) Inhibition of angiogenesis, tumor growth, and metastasis by cathepsin E. Jikken Igaku Zokan. Jan; 22 (2) 213-219.
2. Yamamoto K and Iwata J (2003) Involvement of cathepsin D and cathepsin E in the inhibitors of tumor growth and metastasis through the production of angiogenic inhibitors. Tanpakushitsu Kakusan Koso. Protein, nucleic acid, enzyme, Nov; 48 (14) 1928-33.
News
Featured
Recent News
Lab Photos
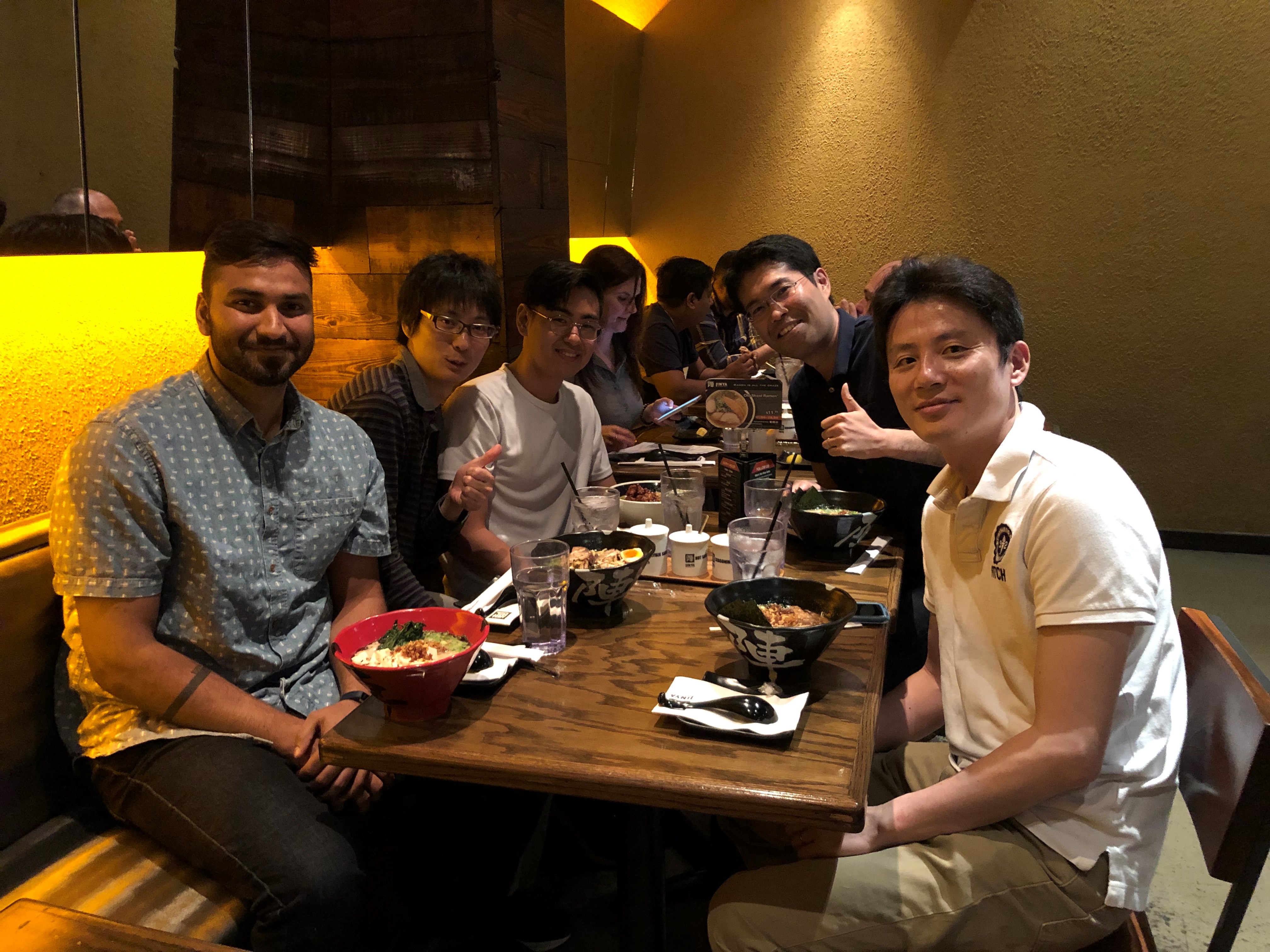
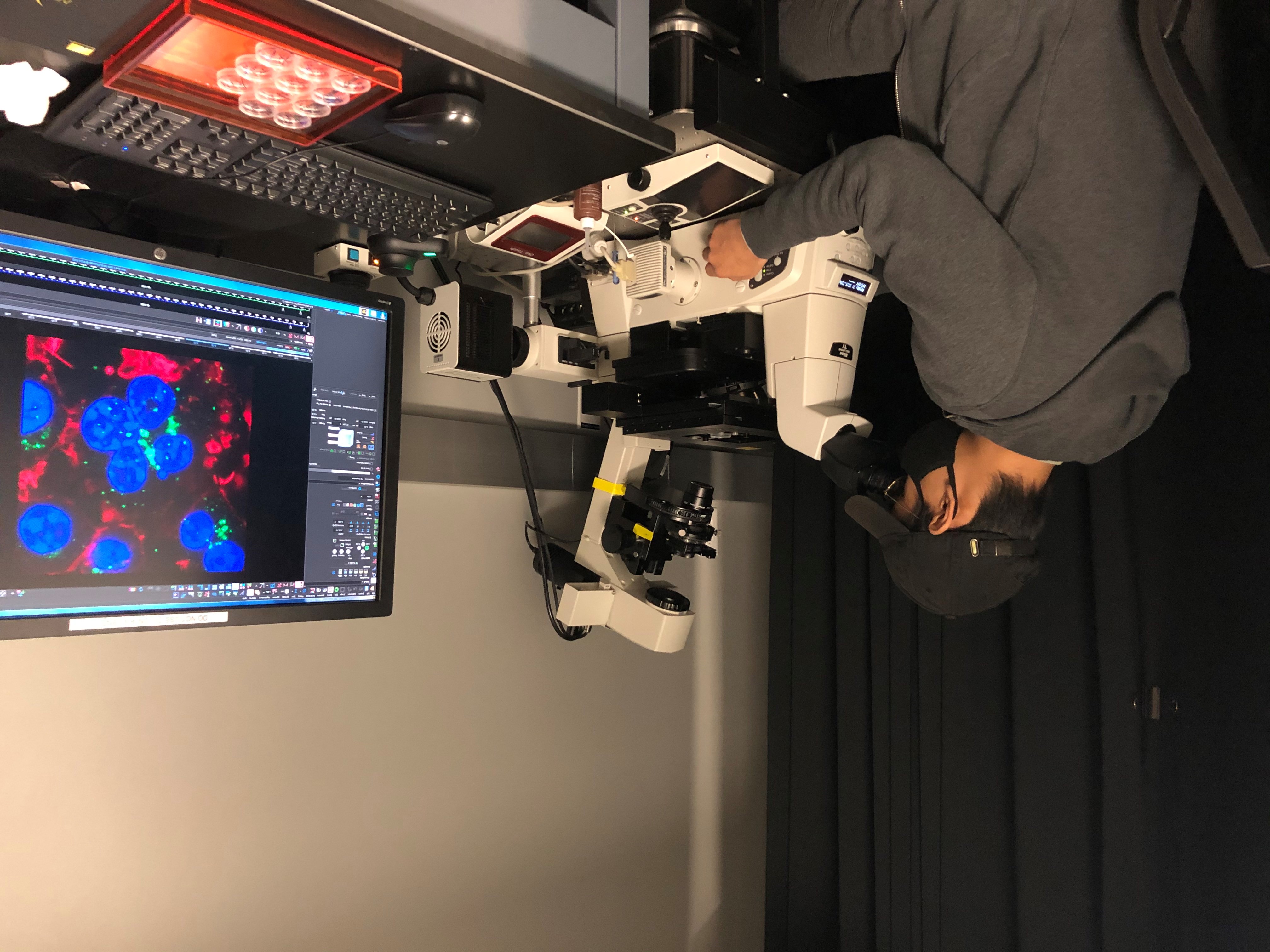
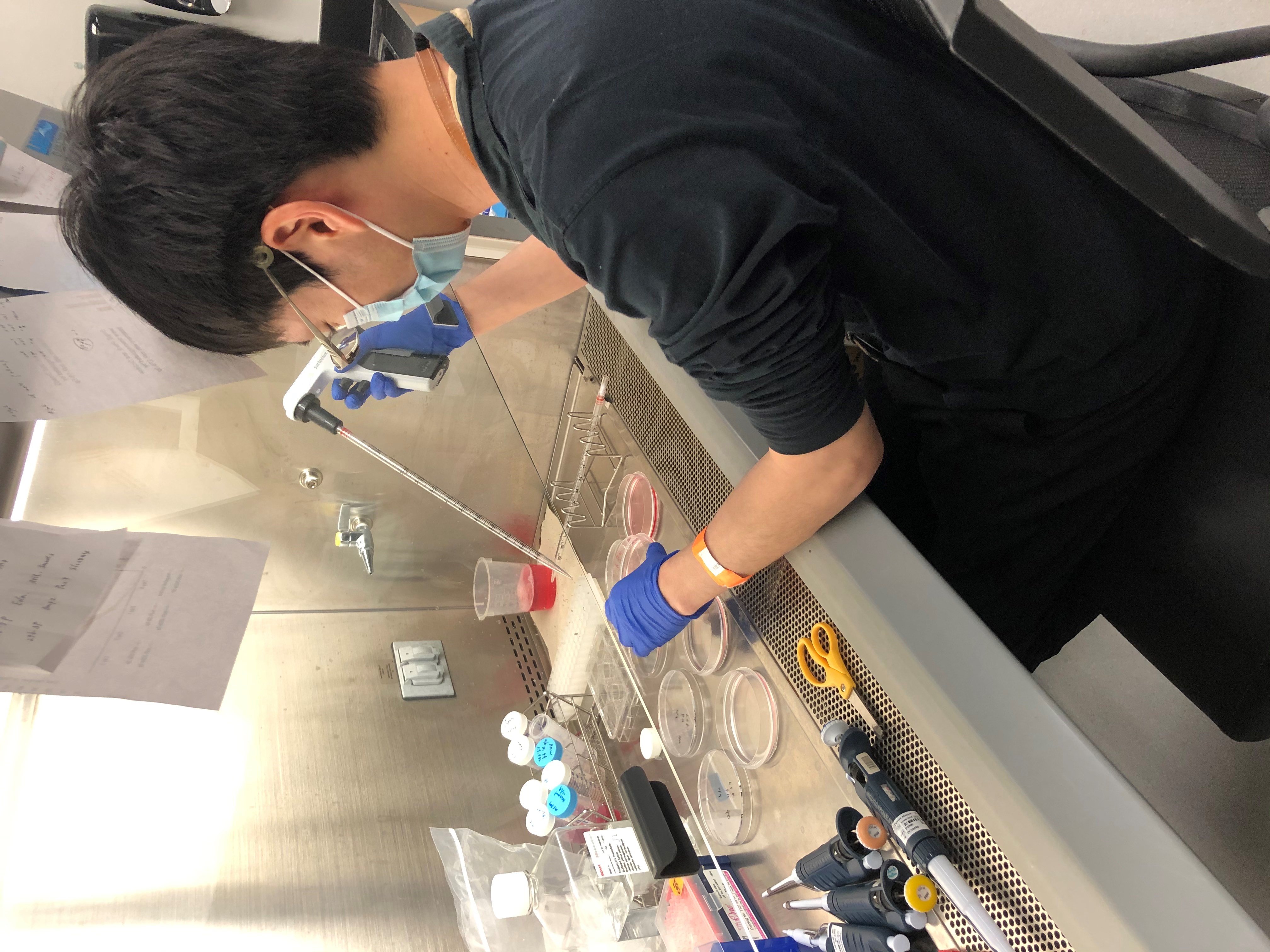